While these approaches yield valuable access to brain activity, they are limited in their temporal and spatial resolution and provide little insight into causal brain mechanisms underlying behavior.
By contrast, neuroscientists working with rodents or even nonhuman primates have access to extremely powerful data collection methods that provide striking insights into the function of molecules, cells, and circuits in the brain. In this case, the limitations come at the expense of using animal models whose behavioral and cognitive abilities differ from those of humans and are without diseases that are inherently human.
Therefore, it would be extremely valuable to apply the power and resolution of more invasive recording techniques that were developed in rodent and nonhuman primate systems to the study of sophisticated human cognition and human disease.
While this is difficult, there are situations when such methodologies can be applied to humans. They include leveraging invasive clinical interventions when patients undergo neurosurgery for diagnosis or treatment of a range of brain disorders, including epilepsy and Parkinson’s disease. Such interventions require the implantation of electrodes deep into the brains of these patients, which permits the direct recording of electrophysiological activity at the source.
Electrode recordings in such patients provide a unique opportunity to measure neural activity in highly localized deep brain structures and thereby help inform our understanding of brain function and dysfunction. In the clinic, the resulting data are extremely valuable to physicians for clinical purposes—including determining the best treatment options for each patient. These unique recordings can also provide an unprecedented view of human brain activity in awake, behaving individuals that is otherwise inaccessible, and guide researchers to generate novel and powerful insights into how the human brain works in health and disease.
Recent studies from the laboratory of Ignacio Saez, PhD, have provided new details into the...
... neurophysiological basis of human cognition from invasive recordings in epilepsy patients.
By leveraging these clinically invasive interventions, neuroscientists are gaining new insights into the function of brain areas, brain circuits, neurotransmitters, and even individual neurons at an unprecedented level of detail and resolution. This experimental approach has grown over the last decade, boosted by the increased application of these invasive procedures, given their safety, utility, and effectiveness in the clinic.
The nascent field of invasive cognitive neuroscience is starting to make striking observations on the neural basis of human cognition, including memory, language, decision-making, emotion, and social behavior—processes that are unique or particularly relevant to human beings. The Nash Family Center for Advanced Circuit Therapeutics and Founding Director Helen S. Mayberg, MD, are at the forefront of these efforts.
Recent studies from the laboratory of Ignacio Saez, PhD, have provided new details into the neurophysiological basis of human cognition from invasive recordings in epilepsy patients. These insights have demonstrated high-frequency activity in human orbitofrontal cortex, a key region for making value-based decisions. The findings suggest that this brain region encodes reward-relevant information, including expected reward and risk associated with uncertain choices. (See Figure 1.)
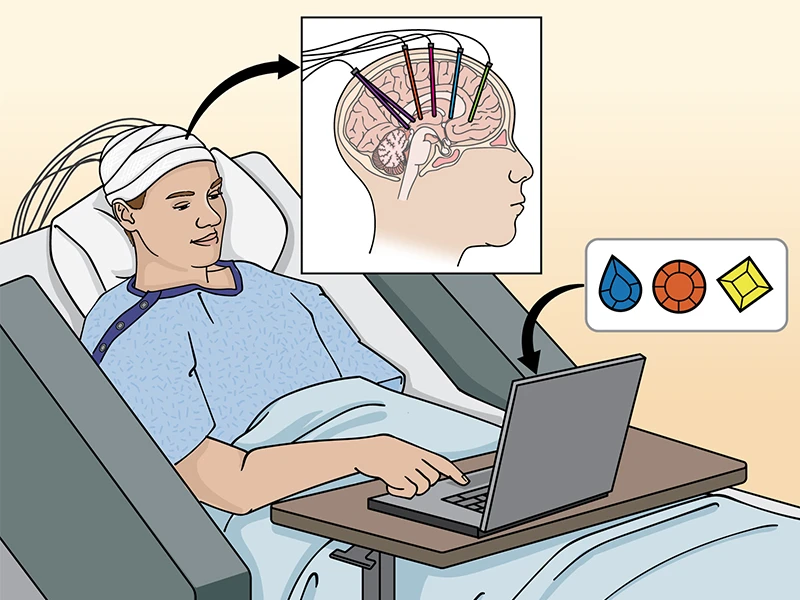
Figure 1. Neurosurgical approaches to the study of human cognition. Epilepsy patients undergo invasive monitoring for the localization of seizure onset zones, which requires implantation of electrodes capable of monitoring brain electrical activity in multiple brain areas. Combined with behavioral tasks and computational models, this allows for direct investigation of the neurobiological basis of human behavior and cognition. By having monkeys perform in some cases the identical cognitive tasks (see related article) it is possible to move bidirectionally from humans to animals and back to humans to establish causal mechanisms. Credit: J Gregory, Mount Sinai Health System
This region also encodes socially relevant information about the rewards obtained by others. The ability to sample brain activity in epilepsy patients, including multiple cortical areas (orbitofrontal, anterior cingulate, and lateral prefrontal cortex) and subcortical areas (amygdala and hippocampus), has provided valuable evidence for the distributed nature of decision-making in the human brain. Remarkably, our findings also suggest that future decisions can be predicted based on patterns of neural activity, which paves the way for machine learning and other forms of artificial intelligence to decode cognitive brain states in humans with debilitating brain disorders and thereby assist efforts at brain-computer interfaces that will aid in their treatment (learn more here).
Other invasive studies provide even greater neurobiological detail, for example, allowing direct measurement of the activity of individual neurons or concentration of individual neurotransmitter molecules within discrete regions of a patient’s brain.
By leveraging deep-brain stimulation surgeries in Parkinson’s disease patients, a recent study provided new evidence for the computational role of individual neurons in the substantia nigra during decision-making, demonstrating that the firing activity of individual putative dopaminergic neurons reflects the reward expectations that guide behavior.
Likewise, recent efforts from the laboratory of Xiaosi Gu, PhD,who directs the Center for Computational Psychiatry, working in concert with Dr. Mayberg, have begun to unveil the relationship between sub-second neurotransmitter dynamics and human cognition and disease by applying fast-scan cyclic voltammetry techniques to directly measure dopamine and serotonin concentrations during deep-brain stimulation surgeries for treatment-resistant depression.
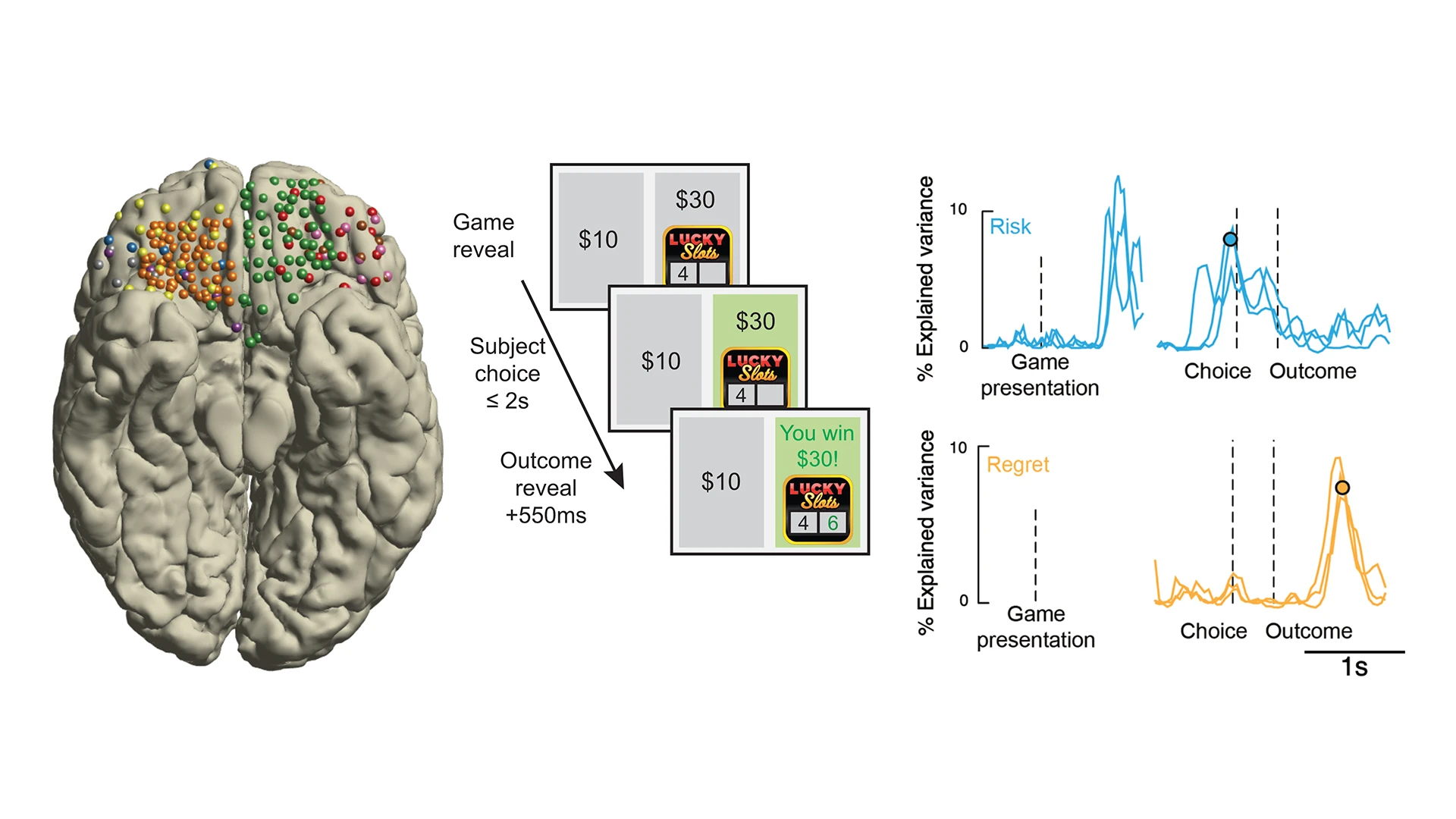
Figure 2. Unveiling the electrophysiological basis of reward decisions. Electrophysiological recordings from the orbitofrontal cortex (left: each color represents recordings from a different patient) in combination with simple decision-making games (middle: patients play a gambling task in which they choose between a safe bet and a risky gamble for a higher reward) reveal the nature of information reflected in brain activity. During decision-making under uncertainty, high-frequency brain electrical activity in orbitofrontal cortex reflects information relevant to ongoing and future decisions, such as the risk of the options currently during deliberation (right, top, blue traces) or the regret experienced after learning a choice was erred (right, bottom, orange traces).
In addition to providing this unique level of neurobiological detail through passive recording of neural activity, invasive approaches provide the ability to carry out neuromodulation to begin to establish causal mechanisms in humans.
This provides two significant opportunities. First, it allows for directly evaluating the involvement of individual brain areas in behavior by examining the behavioral consequences of targeted stimulation. This provides powerful new insights that go beyond correlation, for example, activity in orbitofrontal cortex is associated with decisions, and into causation, for example, is activity in orbitofrontal cortex causing certain types of decisions. Second, it opens the door to the discovery of new treatments for neurological and psychiatric disorders. Invasive neuromodulation strategies are already widely used to treat some disorders, such as movement disorders and epilepsy, and show increasing promise for application to others, including treatment-resistant depression and obsessive-compulsive disorder.
At Mount Sinai, close integration between clinical and research efforts are providing the ideal environment to develop a world-leading invasive human cognitive neuroscience program. This work is accompanied by complementary efforts in animal models to better understand underlying mechanisms of cognitive processes. Human neurophysiology is also guiding efforts at brain-computer interfaces and multiomics studies of human brain as described in other articles highlighted in this newsletter. Together, these approaches have the ability to yield transformative insights into the unique biology of the human brain.
Featured
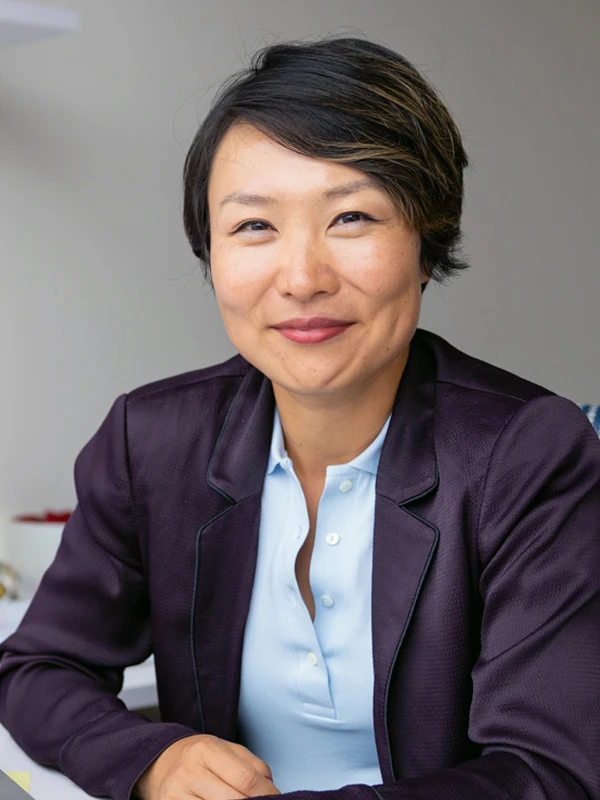
Xiaosi Gu, PhD
Director, Center for Computational Psychiatry, and Associate Professor, Psychiatry, and Neuroscience

Helen S. Mayberg, MD
Founding Director, Nash Family Center for Advanced Circuit Therapeutics, and Professor of Artificial Intelligence and Human Health, Neurosurgery, Neurology, Neuroscience, and Psychiatry
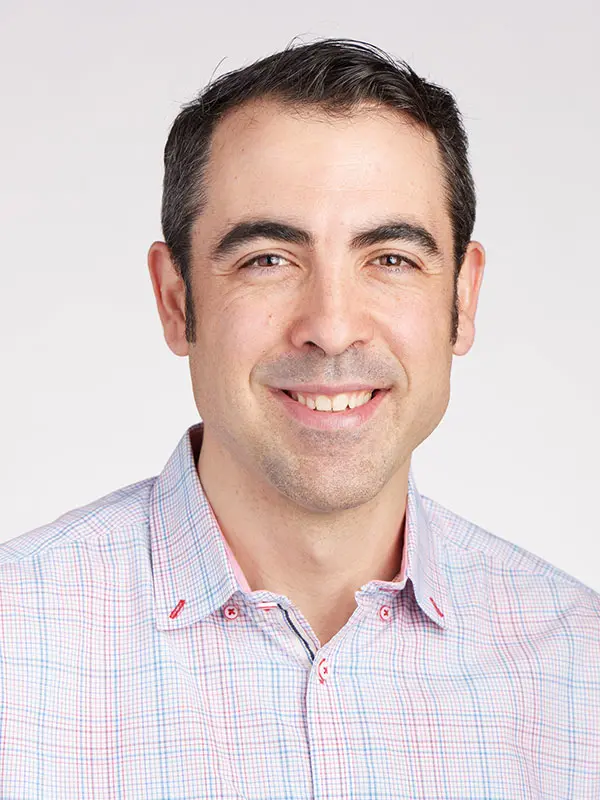
Ignacio Saez, PhD
Associate Professor, Neuroscience, Neurosurgery, and Neurology