Such brain-computer interfaces (BCIs) have been one of the most impactful and paradigm-shifting technologies to emerge from the synergistic scaling of machine learning and electrode microfabrication. This technology now allows us to monitor conscious human thought at a level of detail that has never before been possible.
As clinical trials involving these technologies take shape, Mount Sinai neuroscientists, physicians, and surgeons have been among the first to see and analyze the qualitatively new data. BCIs hold the promise of revolutionizing the diagnosis and treatment of brain disorders.
While information exchange occurs on multiple scales in the human brain, fine motor control, touch sensation, and visual processing, among other functions, are coordinated by populations of neurons spanning submillimeter-scale cortical columns. Partly for this reason, significant attention has been devoted to systems of microelectrodes capable of neural recording or stimulation at corresponding spatial scales.
Whereas neurophysiological recordings from tens of electrode channels were commonplace, with hundreds of channels feasible in the last two decades, the pursuit of high-performance BCIs has given us the capability to build systems with recording capabilities reaching thousands of electrodes—some capable of scaling to tens of thousands of electrodes and beyond—only in the last several years.
This scaling up toward massively multichannel electrode systems is enabling applications in intraoperative neurophysiology and precision neurosurgery, advanced diagnostics and neurocritical care, and neural prosthetics. The operation of such systems requires a paradigm shift in the way electrophysiological data are processed and interpreted, as these BCIs generate such data at scales that are not compatible with human interpretation.
By contrast, large-scale electrode arrays acquire many data streams in parallel, and so lend themselves to the parallel computation architectures so prevalent in modern machine learning hardware systems. Real-time data acquisition, processing, and rendering from contemporary high- channel-count electrophysiological systems have begun employing GPU-accelerated hardware for this reason. As these systems enter mainstream clinical neurophysiology, reliance on artificial intelligence for assistance in electrophysiological data interpretation and diagnosis seems inevitable.
We have had early experience with some of this technology in the intraoperative setting over the last several months. In a pilot human study led by Ignacio Saez, PhD, and Joshua B. Bederson, MD, in collaboration with Saadi Ghatan, MD, and Fedor (Ted) Panov, MD, teams at Mount Sinai have used a high-resolution BCI system developed by Precision Neuroscience to map functional brain regions in exquisite detail. Precision Neuroscience was co-founded by Benjamin Rapoport, MD, PhD, a practicing neurosurgeon in Mount Sinai’s Department of Neurosurgery who has a PhD in electrical engineering and computer science.
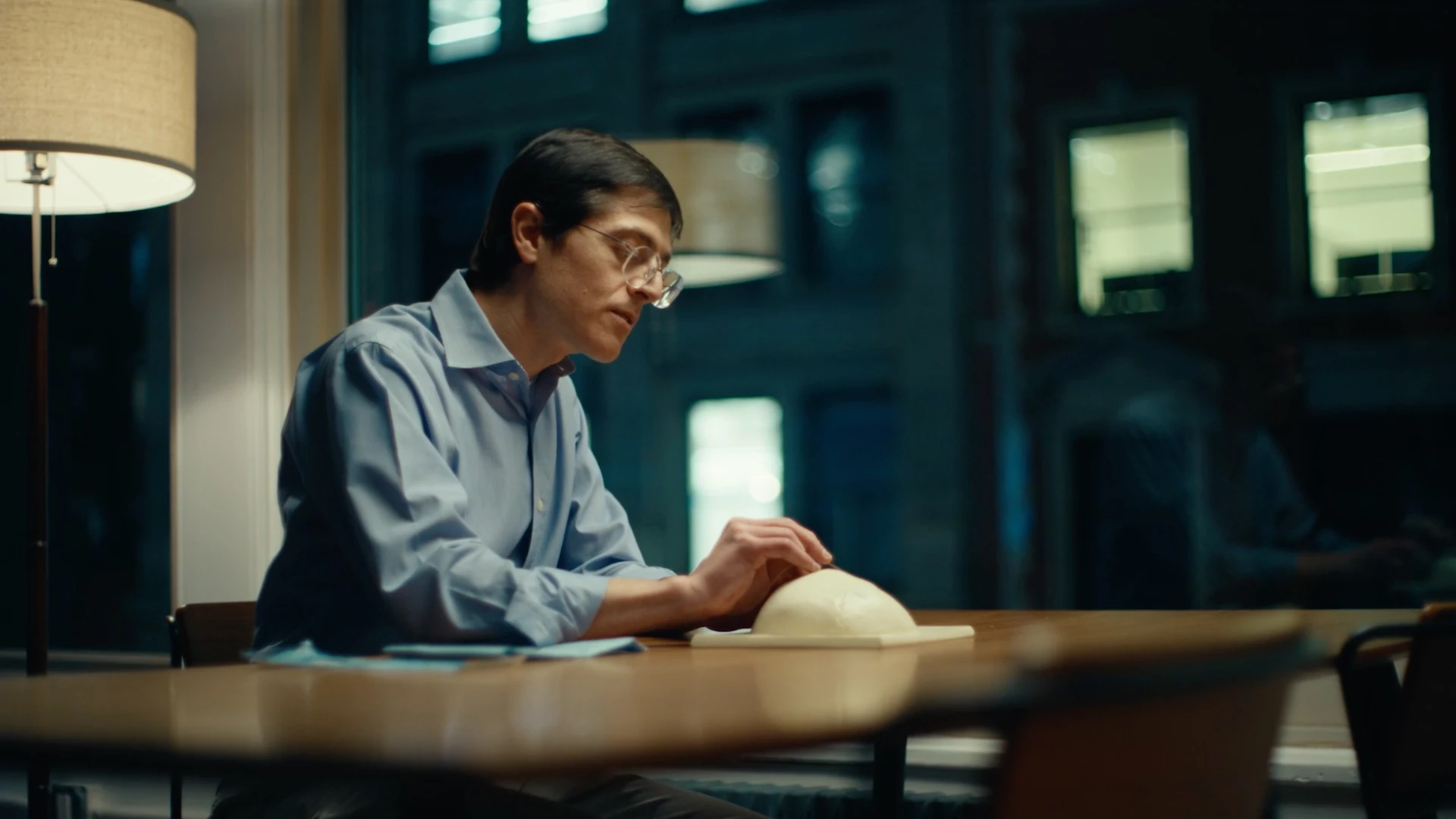
Benjamin Rapoport, MD, PhD
For example, the work has begun to resolve sensory function at the individual finger level on the primary somatosensory cortex (scroll for composite and individual figures), and to map language-related regions in detail during awake epilepsy surgery. In early 2024, this study set a record for deploying the largest number of electrodes—4,096—ever placed on the human brain.
Neural prosthetics are systems that decode motor intention from cortical activity in paralyzed individuals to restore their ability for motoric responses, such as to engage with digital technologies. These prosthetics rely on forms of machine learning to implement the "neural decoding" operations that transform aggregate neural activity from many electrodes into command signals for digital devices. The systems have already begun to enable qualitatively new diagnostic capabilities as well as restoration of function in disease states, such as paralysis, that were previously considered untreatable.
Over the past few decades, numerous independent case reports of several BCI devices have successfully demonstrated the ability to decode speech and motor cortex to control prosthetic limbs, exoskeletons, and digital devices. Although these achievements are striking and promising, due to limitations that include device longevity, practicality, and the invasiveness of the surgery, BCI has yet to progress to any sort of routine clinical applications or into the commercial domain.
Recently, it has become possible to implant special electrodes via transvenous access without the need for invasive brain surgery. These stent-guided electrodes, known as Stentrodes, can be implanted into blood vessels in the brain, such as the superior sagittal sinus, which is adjacent to the primary motor cortex.
Such endovascular BCI technology has emerged as a revolutionary solution to provide minimally invasive intracranial access. It provides a simplified and completely internalized wireless system that does not require a craniotomy, does not need to be connected to a set of large equipment with a team of engineers operating it, and does not trigger inflammatory responses from the brain that have been shown to reduce signals from intracerebral implants over time.
The first endovascular Stentrode implantation in the nation was successfully performed at the Mount Sinai Health System, the leading center for the COMMAND trial that is evaluating the safety and feasibility of endovascular BCI. This work is being led by Shahram Majidi, MD, and Thomas J. Oxley, MD, PhD, both faculty in Mount Sinai’s Department of Neurosurgery. Dr. Oxley is Co-Founder and Chief Executive Officer of Synchron, the manufacturer of the Stentrode.
These various technological innovations are advancing rapidly and promise a fundamentally new approach both to understand how the human brain functions—how it perceives the world and plans and executes responses—and to help individuals with severe brain diseases or injury recover lost function. The work also illustrates how the neurosurgical suite has become a human brain laboratory that promises transformational progress in neuroscience.
The electrodes described here represent an investigational device that is not currently available for sale in the United States. Scroll below for images that illustrate intraoperative mapping of primary somatosensory cortex at high resolution using 2,048 surface microelectrodes.
Dr. Bederson is Chair for the Department of Neurosurgery at the Mount Sinai Health System. Drs. Rapoport and Oxley are faculty members in the Department of Neurosurgery. Dr. Bederson serves in a supervisory role for them within the Health System. Dr. Rapoport is an equity owner in Precision Neuroscience, serves as its Chief Science Officer, and is a member of its board of directors. Neither Dr. Bederson nor Mount Sinai has a financial interest in Precision Neuroscience. Dr. Oxley is an equity owner in Synchron, serves as its Chief Executive Officer, and is a member of its board of directors. Dr. Bederson and other faculty members at Mount Sinai are equity owners in Synchron. All Precision Neuroscience and Synchron research at Mount Sinai is conducted by investigators without financial ties to either company.
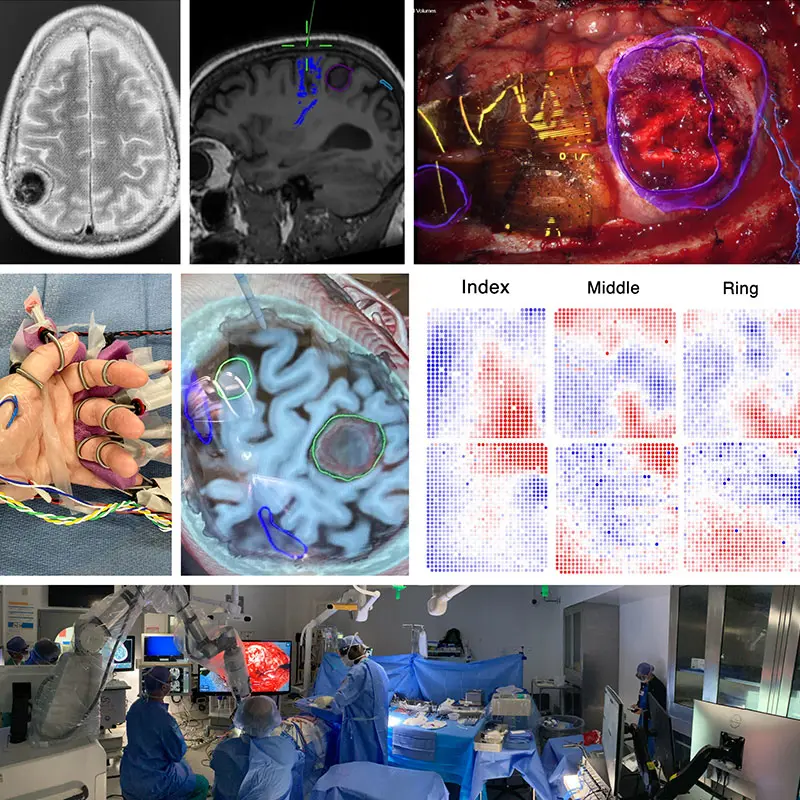
Figure 1. This composite figure illustrates intraoperative mapping of primary somatosensory cortex at high resolution using 2,048 surface microelectrodes.
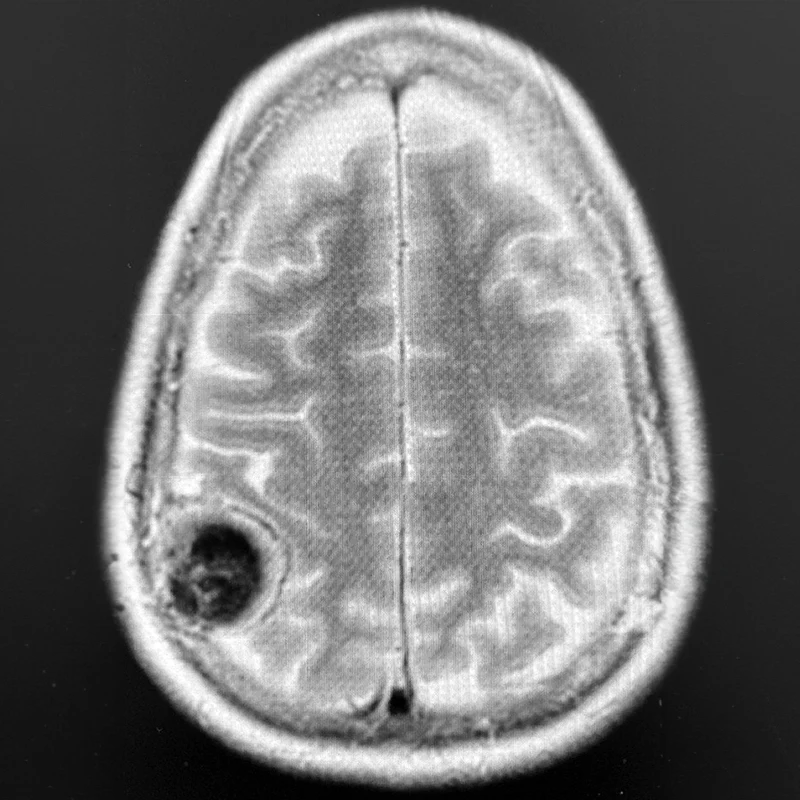
Top Left. Axial T2-weighted brain MRI showing a 4-cm right parietal meningioma compressing the postcentral gyrus (hand and arm primary somatosensory cortex) in a patient who originally presented with a seizure involving sensory and motor changes on the left side of the body.
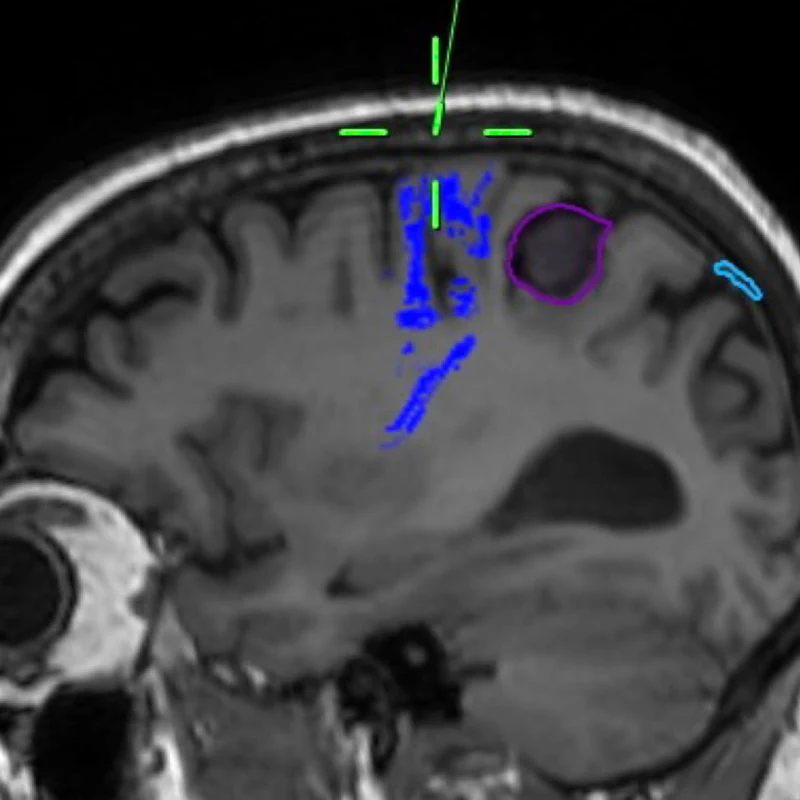
Top Center. Sagittal T1-weighted brain MRI with tractography sequences based on diffusion-weighted imaging techniques. The corticospinal tracts descending from primary motor cortex are highlighted in blue, anterior to the tumor.
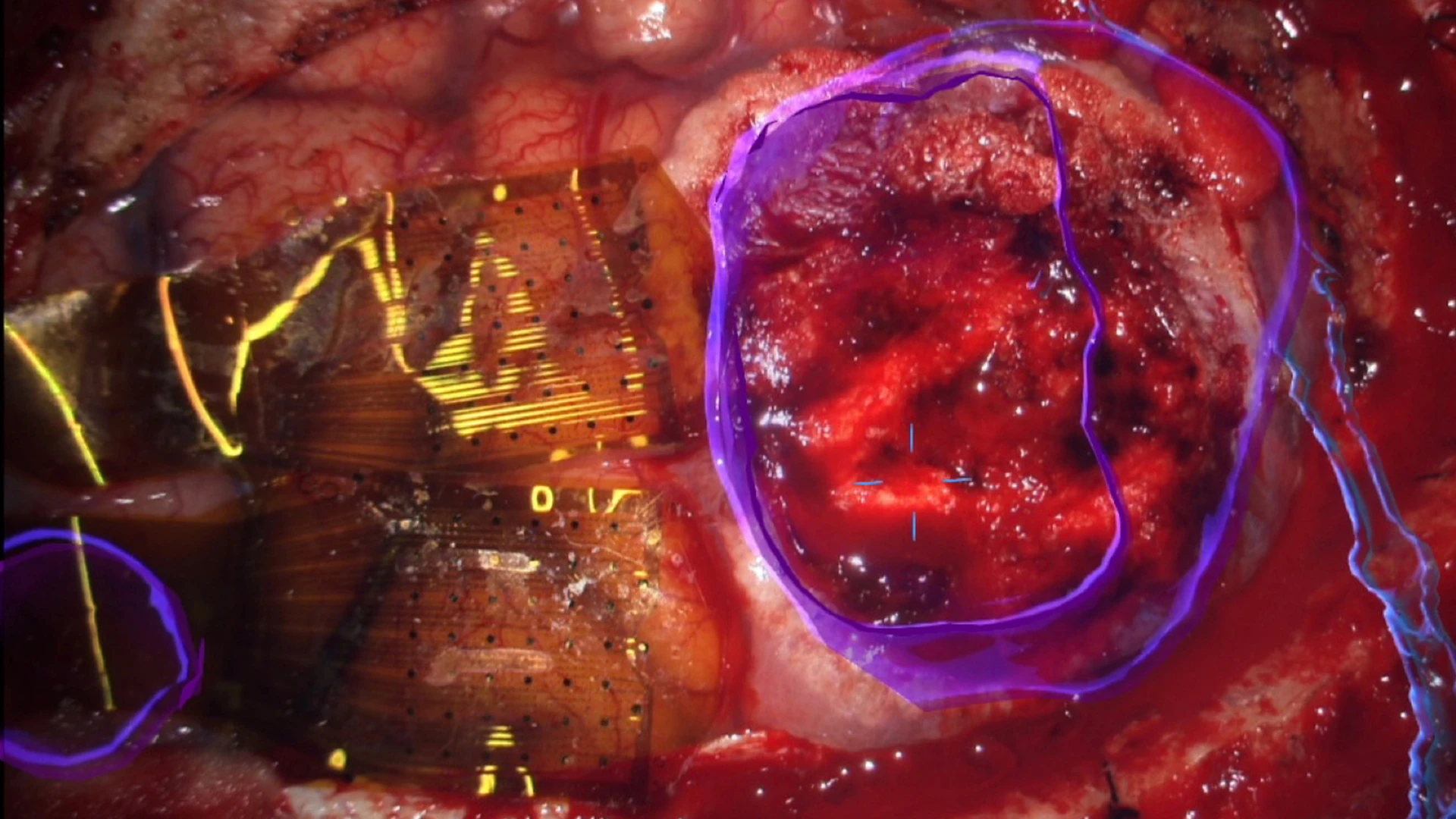
Top Right. Operative field as seen through an operative microscope, with augmented reality overlay in blue to highlight the tumor, right. Two 1,024-electrode arrays, totaling 2,048 microelectrodes, are shown in position straddling the central sulcus, including the precentral (motor) and postcentral (somatosensory) gyri anterior to the tumor, left.
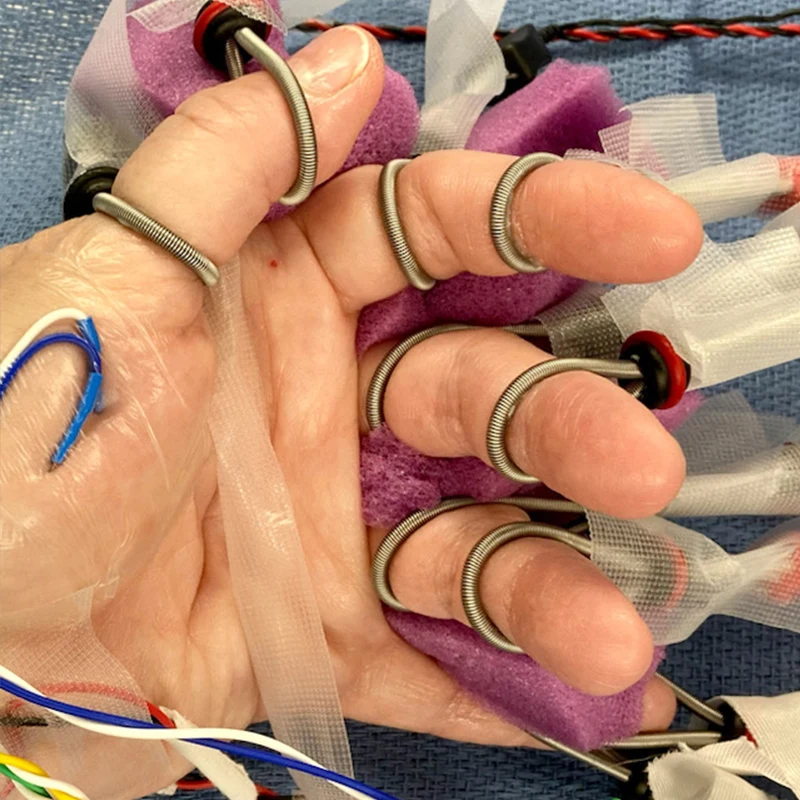
Middle Left. Left hand of the patient with bipolar ring-type stimulating electrodes on each finger to enable synchronized finger-level electrical stimulation and to identify the corresponding finger-specific somatosensory-evoked potentials on the cortical surface.
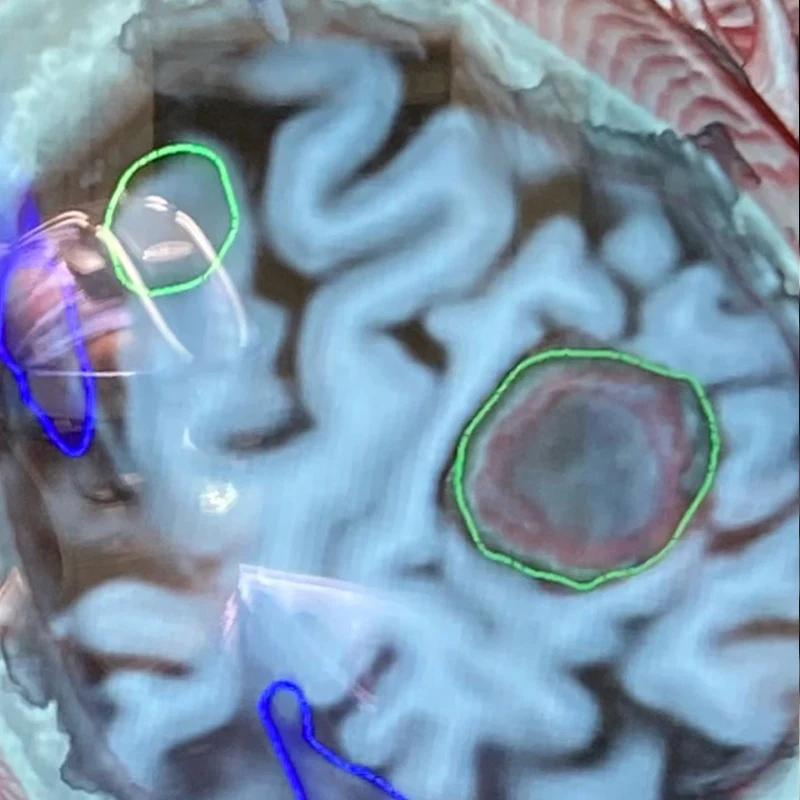
Middle Center. Oblique cross-section through the T1-weighted MRI highlighting the tumor, green, right, compressing the postcentral (sensory) gyrus.
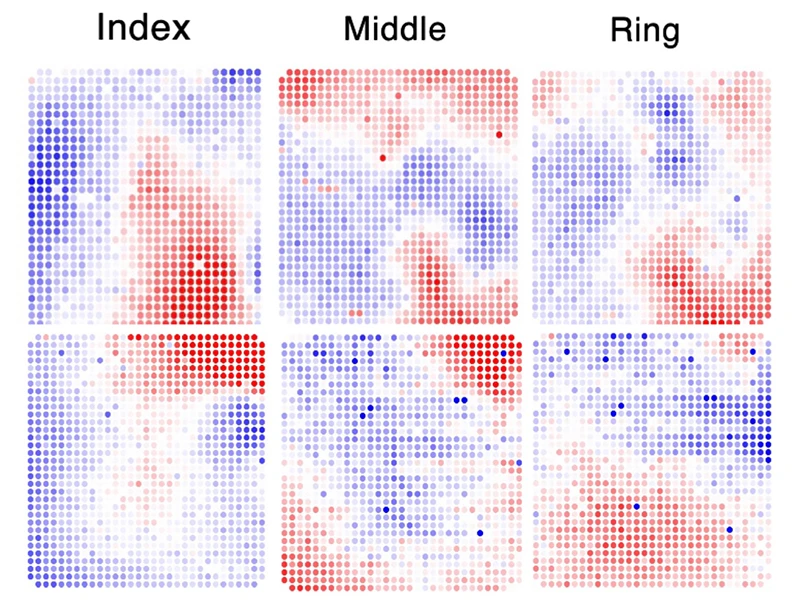
Middle Right. Somatosensory evoked potentials corresponding to individual finger stimulation confirm overlapping but distinguishable regions of primary somatosensory cortex most responsive to stimulation of the index, middle, and ring fingers. Red represents positive surface voltage, blue represents negative voltage. Each dot represents a single 50 µm electrode, with inter-electrode spacing of 400 µm. The arrays are positioned as shown in the intraoperative image in top right.
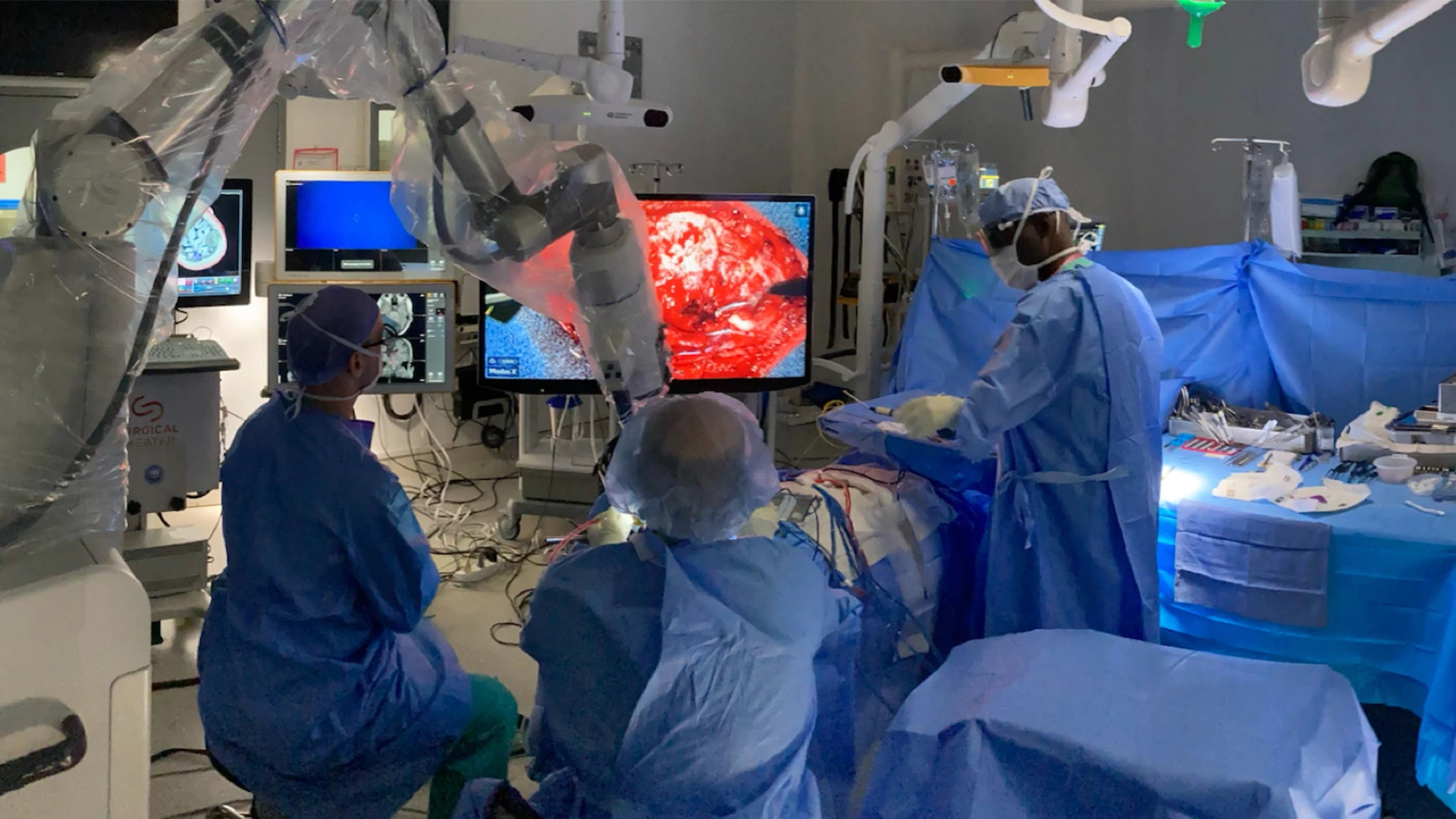
Bottom. Intraoperative setup including displays for frameless stereotactic neuronavigation, left; augmented reality overlay, 3D rendering of the operative field, and high-resolution electrocorticography by Precision Neuroscience, far right. Neurosurgeon Joshua B. Bederson, MD, is positioned at head of patient.
Featured
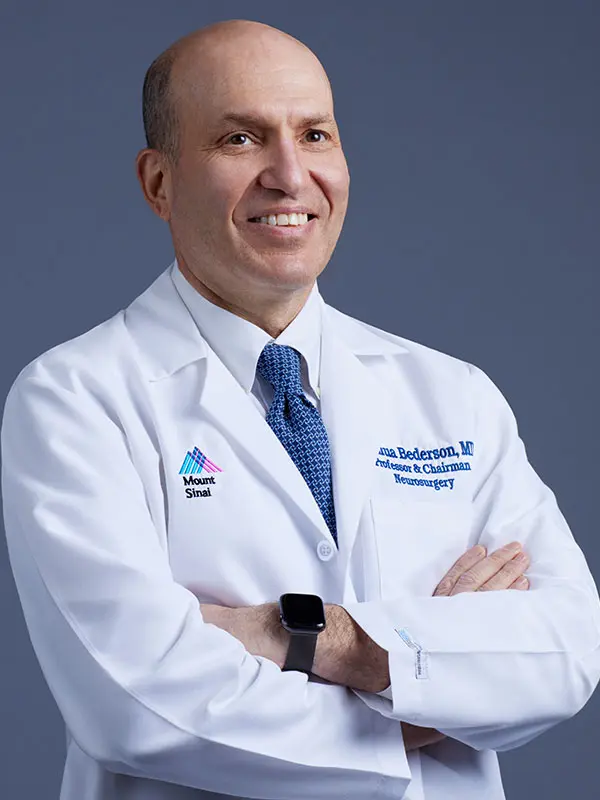
Joshua B. Bederson, MD
Leonard I. Malis, MD/Corinne and Joseph Graber Professor of Neurosurgery and Chair, Department of Neurosurgery at the Mount Sinai Health System
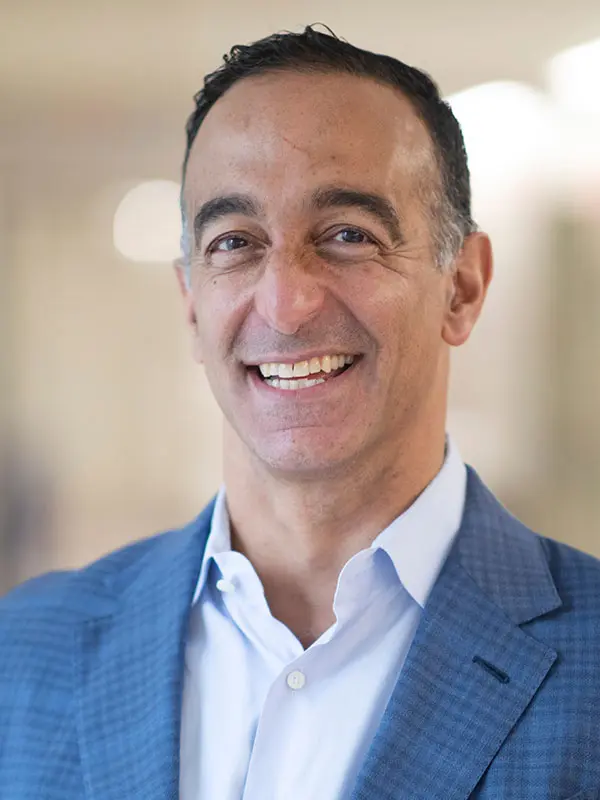
Saadi Ghatan, MD
Professor of Neurosurgery, and Pediatrics, and Chair of Neurosurgery, Mount Sinai West, and Mount Sinai Morningside
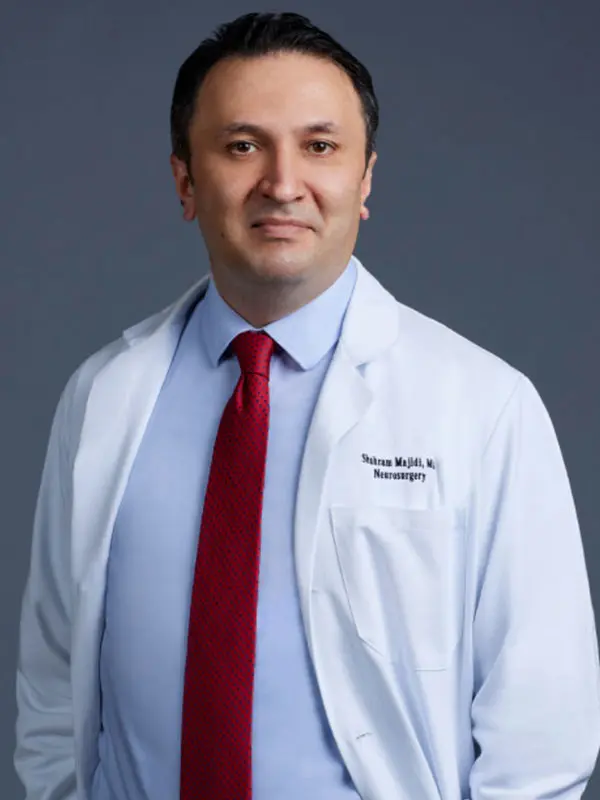
Shahram Majidi, MD
Assistant Professor, Neurosurgery, Neurology, and Diagnostic, Molecular and Interventional Radiology
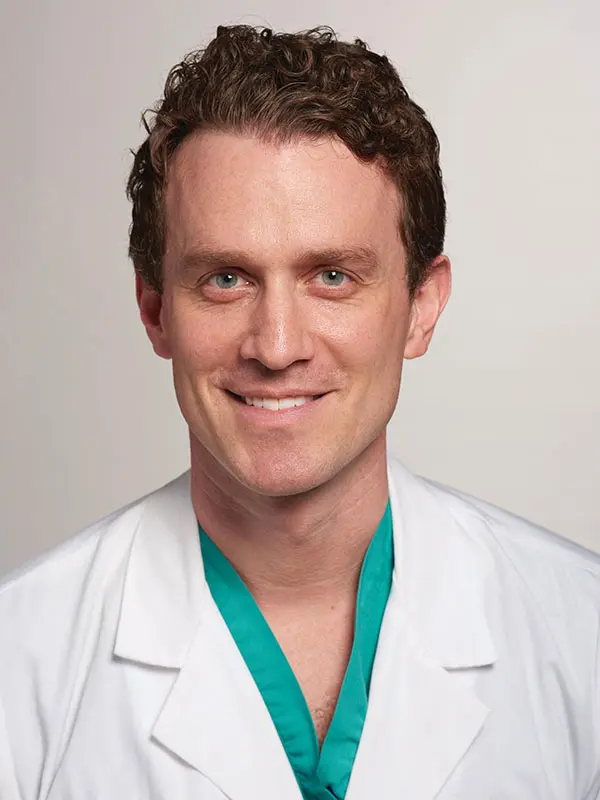
Thomas J. Oxley, MD, PhD
Instructor, and Director of Intervention Strategy, Department of Neurosurgery, Mount Sinai Health System
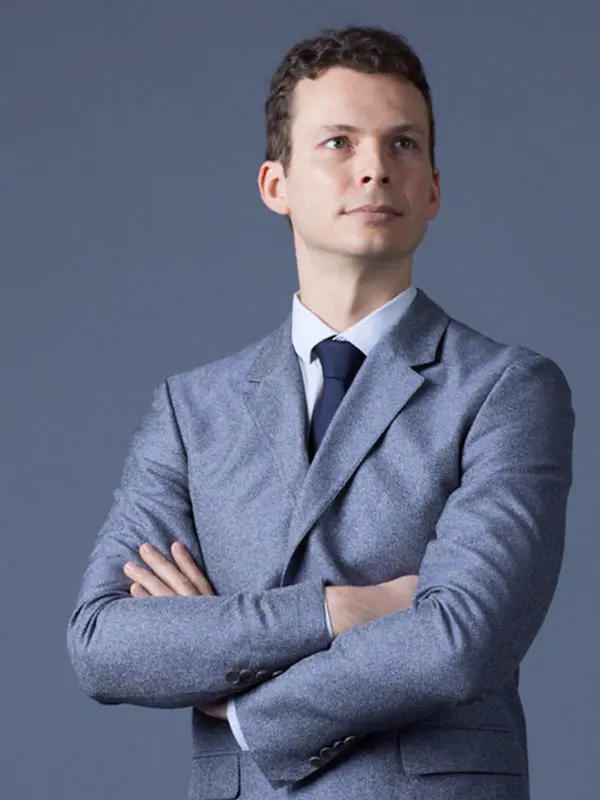
Fedor (Ted) Panov, MD
Associate Professor, Department of Neurosurgery, and Director, Adult Epilepsy Program, Mount Sinai Health System
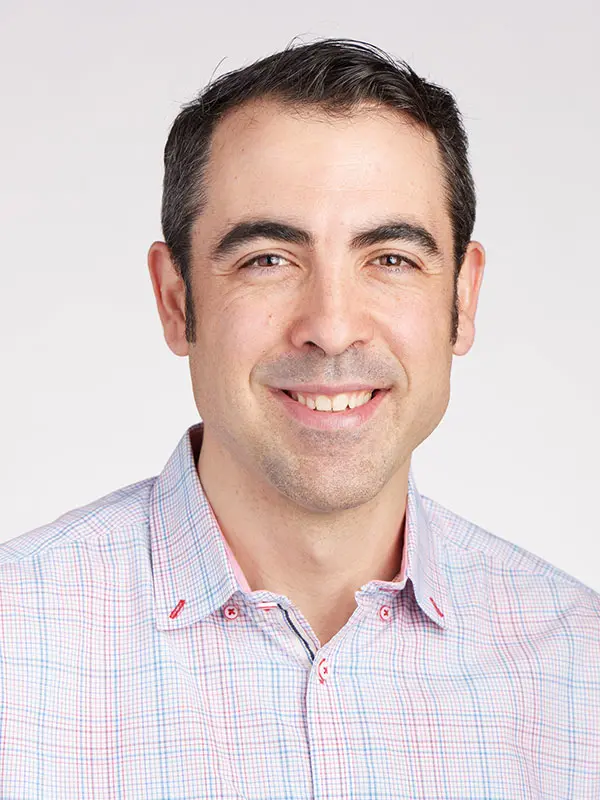
Ignacio Saez, PhD
Associate Professor of Neuroscience, Neurosurgery, and Neurology