The Cardiovascular Research Institute (CVRI) in 2023 continued to advance its discovery and innovation in lifestyle and prevention, systems physiology and bioengineering, and genetic medicine, under the leadership of Director Filip K. Swirski, PhD.
Among the achievements was the renewal of an $11.5 million grant from the National Heart, Lung, and Blood Institute of the National Institutes of Health, funding a multidisciplinary effort led by Zahi Fayad, PhD, to investigate the effects of psychosocial stress on cardiovascular disease. A mechanistic study led by Dr. Swirski, and published in the journal Immunity, found that fasting can trigger a negative effect on monocytes and inflammation in mouse models.
With their teams, CVRI investigators continued to make discoveries in basic and translational science, including development of a modified mRNA translation system for myocardial repair by Lior Zangi, PhD; the study of adeno-associated virus for cardiac gene delivery by Susmita Sahoo, PhD; and experiments on heart cells in the microgravity of the International Space Station by Kevin Costa, PhD. The investigators provide some insights into their work below.
The CVRI is actively hiring faculty and continuing to expand, Dr. Swirski says. “While it is critical to reinforce the values of discipline and hard work, we also support the love of science and creativity,” he says. “It is this positive energy and enthusiasm that we strive to cultivate at the Cardiovascular Research Institute at Mount Sinai as we pursue our mission to prevent, understand, and treat cardiovascular disease.”
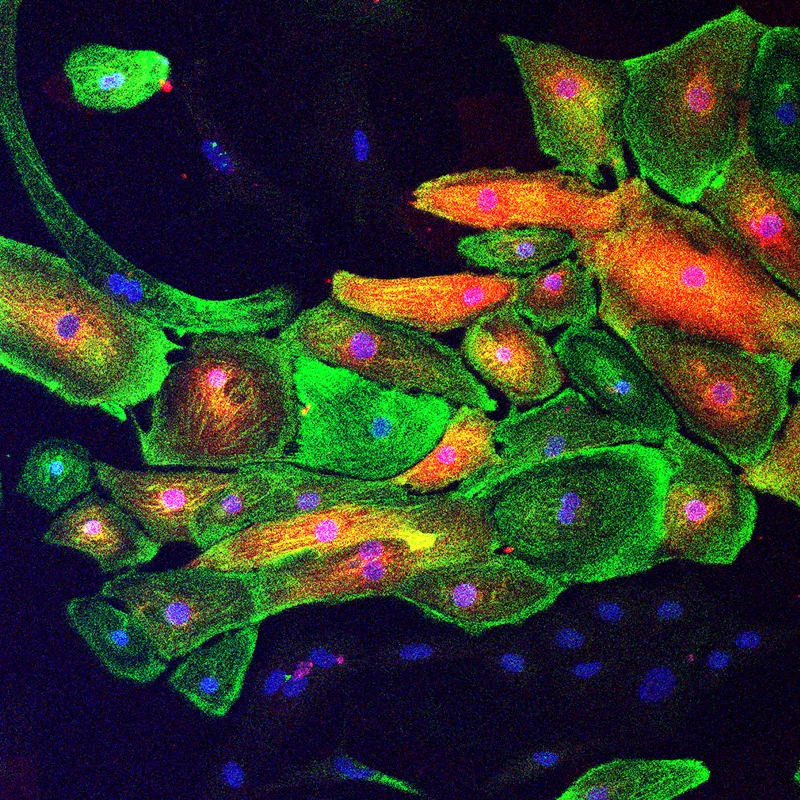
Extracellular vesicle-encapsulated AAVs (EV-AAVs), secreted naturally by AAV-producing cells, are a superior cardiac gene delivery vector that delivers more genes and offers higher antibody resistance. Figure: EV-AAV-luciferase mediates gene expression in iPSC-derived cardiomyocytes (green, cTNT; red, luciferase).
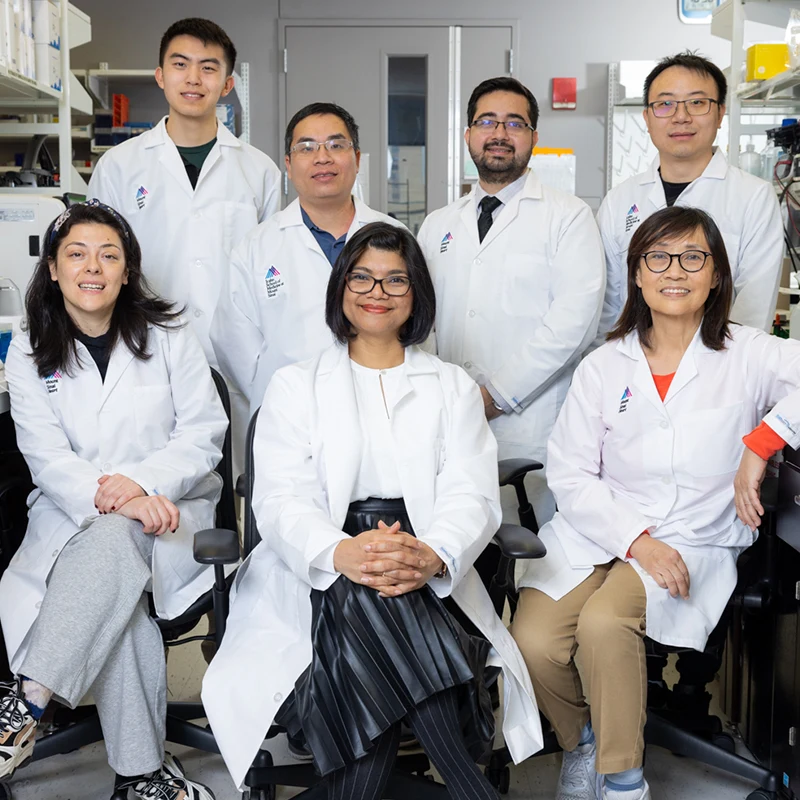
The Sahoo Laboratory. Front row, from left: Sabrina La Salvia, PhD, Susmita Sahoo, PhD, and Shihong Zhang.
Back row, from left: Alan Shao, Anh Phan, PhD, Nikhil Raisinghani, MD, and Xisheng Li, PhD.
Abstract of Study: Extracellular Vesicle Encapsulated Adeno-Associated Viruses for Therapeutic Gene Delivery to the Heart Circulation, August 2023
Adeno-associated virus (AAV) has emerged as one of the best tools for cardiac gene delivery due to its cardiotropism, long-term expression, and safety. However, a significant challenge to its successful clinical use is preexisting neutralizing antibodies (NAbs), which bind to free AAVs, prevent efficient gene transduction, and reduce or negate therapeutic effects. Here, we describe extracellular vesicle-encapsulated AAVs (EV-AAVs), secreted naturally by AAV-producing cells, as a superior cardiac gene delivery vector that delivers more genes and offers higher NAb resistance.
We developed a two-step density-gradient ultracentrifugation method to isolate highly purified EV-AAVs. We compared the gene delivery and therapeutic efficacy of EV-AAVs with an equal titer of free AAVs in the presence of NAbs, both in vitro and in vivo. In addition, we investigated the mechanism of EV-AAV uptake in human left ventricular and human-induced pluripotent stem cell-derived cardiomyocytes in vitro and mouse models in vivo using a combination of biochemical techniques, flow cytometry, and immunofluorescence imaging.
Using cardiotropic AAV serotypes 6 and 9 and several reporter constructs, we demonstrated that EV-AAVs deliver significantly higher quantities of genes than AAVs in the presence of NAbs, both to human left ventricular and human-induced pluripotent stem cell-derived cardiomyocytes in vitro and to mouse hearts in vivo. Intramyocardial delivery of EV-AAV9–sarcoplasmic reticulum calcium ATPase 2a to infarcted hearts in preimmunized mice significantly improved ejection fraction and fractional shortening compared with AAV9–sarcoplasmic reticulum calcium ATPase 2a delivery. These data validated NAb evasion by and therapeutic efficacy of EV-AAV9 vectors. Trafficking studies using human induced pluripotent stem cell–derived cells in vitro and mouse hearts in vivo showed significantly higher expression of EV-AAV6/9–delivered genes in cardiomyocytes compared with noncardiomyocytes, even with comparable cellular uptake. Using cellular subfraction analyses and pH-sensitive dyes, we discovered that EV-AAVs were internalized into acidic endosomal compartments of cardiomyocytes for releasing and acidifying AAVs for their nuclear uptake.
Together, using five different in vitro and in vivo model systems, we demonstrate significantly higher potency and therapeutic efficacy of EV-AAV vectors compared with free AAVs in the presence of NAbs. These results establish the potential of EV-AAV vectors as a gene delivery tool to treat heart failure.
Notes From the Investigator: Susmita Sahoo, PhD, Associate Professor of Medicine (Cardiology)
Adeno-associated viruses (AAVs) are one of the best tools for cardiac gene delivery due to their cardiotropism, long-term expression, and safety in clinics. However, a significant challenge to their successful clinical use is futility caused by pre-existing neutralizing antibodies (NAbs), which bind to free AAVs, prevent efficient gene transduction, and reduce or negate the effects of therapy. AAV-NAbs are seropositive over half the human population worldwide, limiting the number of patients eligible for therapy. The presence of NAbs also limits redosing using AAVs in already treated patients. Therefore, strategies to circumvent NAbs are necessary.
Our recent research, published in 2023 in Circulation, has provided the proof of concept that extracellular vesicle (EV)-encapsulated AAVs (EV-AAVs) shield AAVs from NAbs, enhancing gene delivery and overcoming a key roadblock associated with AAV-mediated gene therapy. EV-AAVs are clinically compatible, superior cardiac gene delivery vectors that can advance current AAV-based therapies for treating cardiovascular diseases.
EV-AAVs are conventionally isolated from the conditioned media of transfected HEK 293T cells using a multistep density-gradient ultracentrifugation method, which is complex, time-consuming, and results in significant loss of the starting material. Thus, it is unsuitable for scalable manufacturing and clinical translation. Moreover, free AAVs are released along with EV-AAVs to the conditioned media of AAV-producing cells. Free AAV contaminations in EV-AAV preparations may reduce effective EV-AAV dosing, reduce their NAb resistance, and may induce unwanted side effects from higher doses of free AAVs. Therefore, EV-AAVs should be isolated with minimum contamination from free AAVs. In addition, the challenges to successfully translating EV-AAVs to clinics are multifold: large-scale sourcing of EV-AAVs; scalable isolation method; efficient clinical-grade production, purity, potency, and characterization; optimization of storage conditions and reproducibility, dosing, and toxicity; bioavailability and cellular uptake mechanisms.
In the next steps of our research, we address these key challenges that impede the use of EV-AAV therapy in clinics. We are currently developing optimal and scalable strategies for production and isolation of EV-AAVs with minimum contamination from free AAVs. We are also developing robust analytics, functional assays, cardiac gene delivery, and cellular uptake mechanisms toward its translation to clinics.
Currently, we are partnering with an exosomes company, Evox Therapeutics, based in the United Kingdom, to use its large-scale manufacturing technology with a 3D-suspension culture, large-scale production of EV-AAVs using a size exclusion chromatography-based isolation method and studying the cardiac delivery and cardiotropism mechanisms of EV-AAVs.
Our studies will yield critical methodological advances and technology development necessary for future translation of EV-AAVs. These key validation points will develop a final therapeutic product toward an innovative solution to a critical barrier in gene therapy and will justify its entry into clinics.
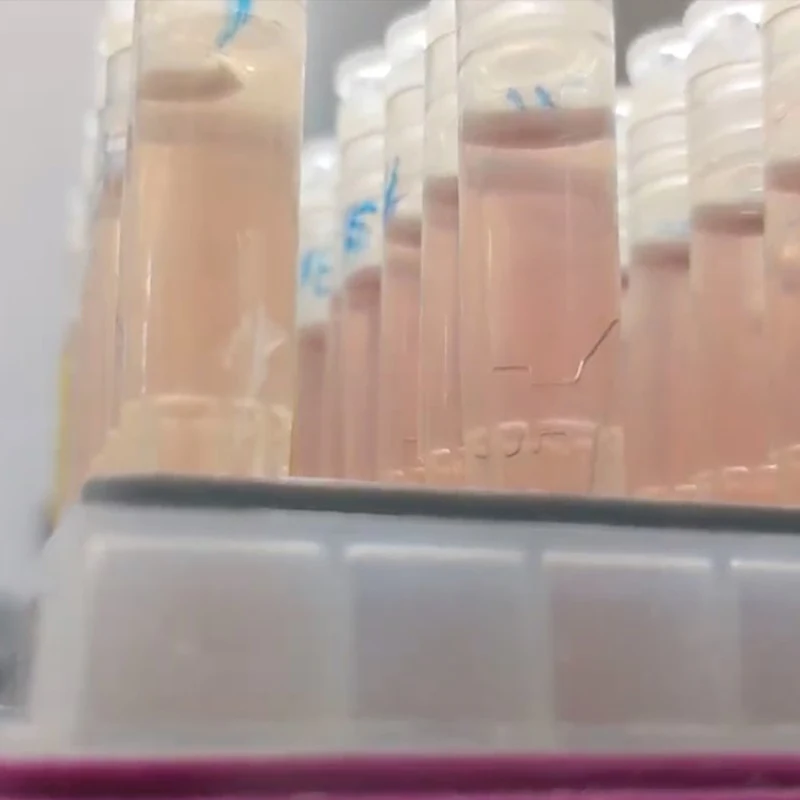
One-milliliter cryovials containing cardiac muscle cells and micro-tissues, after their return from the International Space Station.
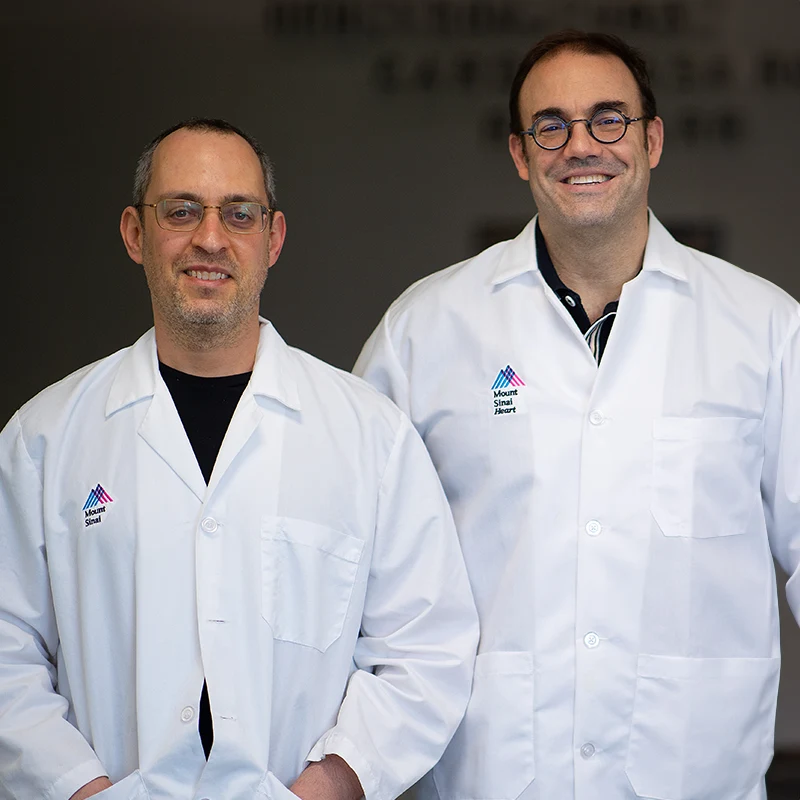
Kevin Costa, PhD, right, and David Sachs, PhD
Study: Cardiac Muscle Samples Sent Into Space to Study Heart Cell Biology in Microgravity
In a project led by Kevin Costa, PhD, the Cardiovascular Research Institute sent bioengineered human heart muscle cells and micro-tissues into space for the first time on NASA’s 29th SpaceX commercial resupply services mission, which launched on November 9, 2023. The mission sent scientific research to the International Space Station (ISS), where the samples stayed 30 days before returning to Earth on December 22, 2023.
Through the experiment, researchers aim to gain a better understanding of how cardiomyocytes adapt to extreme biological stresses, and how microgravity and other features of space travel impact heart cell function. Understanding the capabilities and limitations of such heart cells to survive is not only important for the health of astronauts, but also a first step toward future efforts in space-based tissue engineering, organoid fabrication, and bioprinting, which are all important in the emerging economy of biomanufacturing in the microgravity environment known as low Earth orbit.
Mount Sinai is partnering with the tech company Space Tango, which provides access to microgravity for research and development purposes on the ISS. Space Tango managed Mount Sinai’s tissues, which were sealed in individual small-scale experimental containers called cryovials and placed in a larger containment unit known as a CubeLab. These one-milliliter vials also contained cell culture media and additives to help keep the cardiac muscle cells alive for an extended period, with some beating and some chemically arrested to reduce their metabolism and see if their survival is affected.
The Mount Sinai investigators, including David Sachs, PhD, and MD/PhD candidate Camille Van Neste from the Costa Lab, generated these human heart muscle cell samples from induced pluripotent stem cells from a healthy adult donor. The cells were cultured in three distinct configurations: 2D monolayers, 3D spheroids, and 3D elongated cardiac tissue formats. This tested whether the 3D culture conditions, which are more physiologic than 2D cultures, offer a biological advantage for the heart cells. Once the cargo capsule carrying the research arrived at the ISS, astronauts placed the CubeLab in a dedicated Space Tango facility. After astronauts returned the samples to Earth, Mount Sinai researchers began their analyses.
Initially upon inspection, none of the cardiac samples were beating. However, after opening the vials and allowing them to recover for 24 hours in an incubator, remarkably some of the tissues resumed beating—they had survived the trip, Dr. Costa says. By contrast, none of the tissues that were sealed in vials on Earth regained function after the experiment, suggesting the microgravity not only induced stresses on the tissues, but might also confer some protective benefits. Analysis is ongoing to understand the differences between samples that survived and those that did not, with a second space flight involving more sophisticated experiments planned for the end of 2024.
Notes From the Investigator: Kevin Costa, PhD, Associate Professor of Medicine (Cardiology)
This project is helping us understand the impact of microgravity and space flight on engineered human heart muscle cells and micro-tissues, and will test for the first time how these highly active beating heart muscle cells adapt to a month of exposure to such extreme conditions. As we gain a better understanding of how these engineered cardiac cells and tissues function, we can find new ways to help protect astronauts so they can stay in space longer to complete more in-depth exploratory missions. This will also provide clues about how to better protect the hearts of people on Earth from the detrimental effects of aging and inactivity.
The goal of the experiment is to assess the ability of our engineered cardiac cells and micro-tissues to survive in a sealed environment in microgravity for 30 days, and to compare the survival characteristics to equivalent samples cultured in our laboratory at Mount Sinai’s Cardiovascular Research Institute. We are testing to see if microgravity will alter the cardiomyocyte ability to adapt to this enclosed environment, and to see if there are differences in the biology of the cells that are returned from the ISS. We hope to learn more about the effects of microgravity on human heart cell and tissue biology, and to explore the possibility of performing such studies in a sealed environment that does not require the usual fluidic exchange systems that significantly add to the complexity of doing cell biology in space.
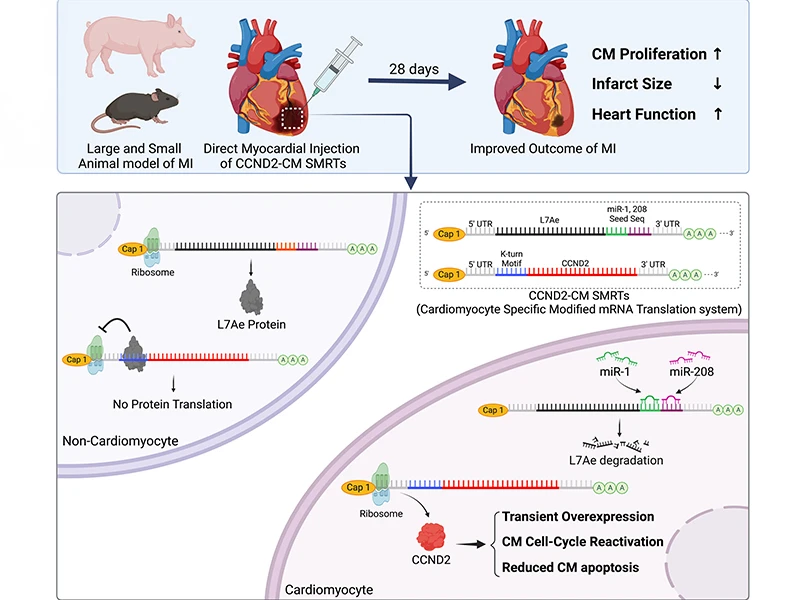
A graphic abstract of the study.
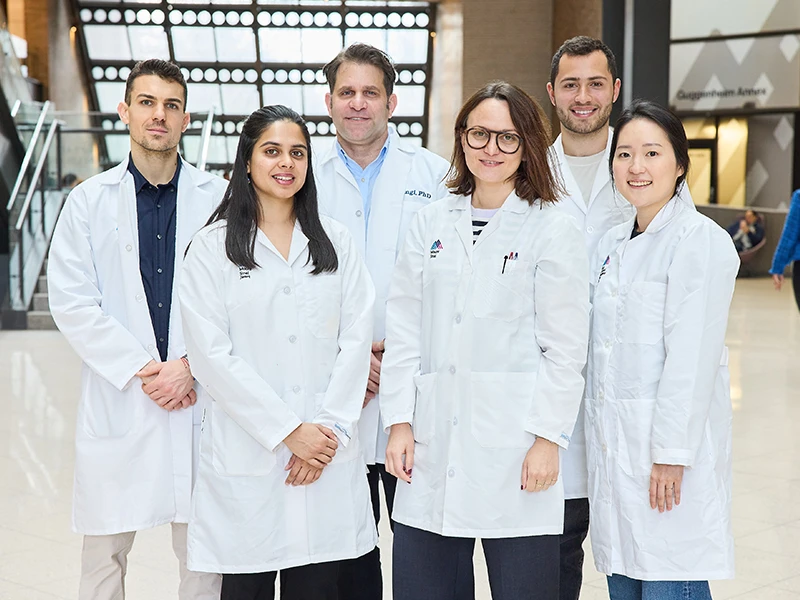
The Zangi Laboratory, from left: Eldar Paz Amir, Gayatri Mainkar, Lior Zangi, PhD, Magdalena Zak, PhD, Matthew Adjmi, MS, and Jimeen Yoo, MS.
Abstract of Study: CCND2 Modified mRNA Activates Cell Cycle of Cardiomyocytes in Hearts With Myocardial Infarction in Mice and Pigs, Circulation Research, August 2023
Experiments in mammalian models of cardiac injury suggest that the cardiomyocyte-specific overexpression of CCND2 (cyclin D2, in humans) improves recovery from myocardial infarction (MI). The primary objective of this investigation was to demonstrate that our specific modified mRNA translation system (SMRTs) can induce CCND2 expression in cardiomyocytes and replicate the benefits observed in other studies of cardiomyocyte-specific CCND2 overexpression for myocardial repair.
The CCND2-cardiomyocyte-specific modified mRNA translation system (cardiomyocyte SMRTs) consists of two modRNA constructs: one codes for CCND2 and contains a binding site for L7Ae, and the other codes for L7Ae and contains recognition elements for the cardiomyocyte-specific microRNAs miR-1 and miR-208. Thus, L7Ae suppresses CCND2 translation in noncardiomyocytes but is itself suppressed by endogenous miR-1 and -208 in cardiomyocytes, thereby facilitating cardiomyocyte-specific CCND2 expression. Experiments were conducted in both mouse and pig models of MI, and control assessments were performed in animals treated with SMRTs coding for the cardiomyocyte-specific expression of luciferase or green fluorescent protein (GFP), in animals treated with L7Ae modRNA alone or with the delivery vehicle, and in sham-operated animals. CCND2 was abundantly expressed in cultured, postmitotic cardiomyocytes two days after transfection with the CCND2-cardiomyocyte SMRTs, and the increase was accompanied by the upregulation of markers for cell-cycle activation and proliferation (e.g., Ki67 and Aurora B kinase). When the GFP-cardiomyocyte SMRTs were intramyocardially injected into infarcted mouse hearts, the GFP signal was observed in cardiomyocytes, but no other cell type.
In both MI models, cardiomyocyte proliferation (on day seven and day three after treatment administration in mice and pigs, respectively) was significantly greater, left-ventricular ejection fractions (days 7 and 28 in mice, days 10 and 28 in pigs) were significantly higher, and infarcts (day 28 in both species) were significantly smaller in animals treated with the CCND2-cardiomyocyte SMRTs than in any other group that underwent MI induction.
Intramyocardial injections of the CCND2-cardiomyocyte SMRTs promoted cardiomyocyte proliferation, reduced infarct size, and improved cardiac performance in small and large mammalian hearts with MI.
Notes From the Investigator: Lior Zangi, PhD, Associate Professor of Medicine (Cardiology), and Genetics and Genomic Sciences
After ischemic cardiac injury, nondividing cardiomyocytes (CMs) die and are replaced by fibrosis and scarring that limit heart function and trigger heart failure, which is the leading cause of death worldwide. To reduce the detrimental effects of ischemic cardiac injury, several strategies—such as tissue engineering, cell therapy, and gene therapy—have been evaluated, with limited success.
Gene therapies include viral (e.g., adeno-associated virus [AAV]) and nonviral (e.g., siRNA, shRNA, and mRNA) gene delivery methods. Modified mRNA (modRNA) is a nonviral gene delivery platform that was used to create the two successful modRNA COVID-19 vaccines, an advance for which Katalin Karikó, MD, and Drew Weissman, MD, PhD, won the Nobel Prize in Medicine. Supporting their discovery, the Zangi Lab’s studies indicate that modRNA also has therapeutic potential for ischemic diseases. Replacing 100 percent of uridine with N1-methylpseudouridine-5'-triphosphate in modRNA synthesis renders it non-immunogenic, with higher translation and RNase endurance than uridine-based mRNA in cardiac cells and tissue. ModRNA delivery in vivo immediately induces high protein expression that lasts for up to 10 days in several cardiac cell types, including CMs. To date, modRNA technology has been used in ischemic heart disease mouse models to promote cardioprotection, CM proliferation for cardiac regeneration, cardiovascular restoration, and attenuation of cardiac fibrosis and hypertrophy post ischemic injury.
To create new cardiac muscle, nondividing CMs need to proliferate. Reactivating CM proliferation has been a key element in fetal or newborn mammals’ cardiac regeneration. Several genes, such as Cyclin D2 (CCND2), are known to promote the cell cycle, including in CMs. As modRNA may translate in all cell types in the heart, CCND2 modRNA delivery will lead to expansion of all cardiac cells and not exclusively CMs. Such non-CM proliferation may increase post-injury fibrosis and inflammation, which are detrimental to the heart. There is therefore a need to generate modRNA that will allow gene delivery specifically into CMs. To prevent modRNA from activating cell types other than CMs, Dr. Zangi designed a CM-specific modRNA translational system (CM SMRTs) that allows modRNA translation preferentially in CMs.
Recent work led by Dr. Zangi and Jianyi “Jay” Zhang, MD, PhD, from the University of Alabama at Birmingham, and published in Circulation Research, showed that CCND2CM SMRTs allows specific translation of CCND2 in CMs but not non-CMs when directly injected into the hearts of mice or pigs. Also, a single treatment of CCND2CM SMRTs, immediately after injury, led to significantly improvement in cardiac function and reduced cardiac scar formation, without any additional risk of arrhythmia in both mice and pig ischemic injury models.
It is worth noting that this study employed CCND2CM SMRTs delivery via open-chest surgery immediately after injury. A more clinically relevant approach needs to deliver CCND2CM SMRTs into the heart without the need for open-chest surgery. The Zangi and Zhang labs are now examining catheter-based CM SMRTs delivery to the heart. Also, it will be important to evaluate the therapeutic windows for this treatment, especially to determine the effects of CCND2CM SMRTs treatment when administered either one or four weeks post ischemic injury. Such inquiries may shed light on the therapeutic promise of using CCND2 CM SMRTs to not only prevent heart failure, but also treat it.