For example:
The advent of radioactively labeled compounds in the early 1970s led to the identification of GPCRs in the brain and facilitated the discovery of novel compounds that could bind to these receptors.
Starting in the late 1980s, the revolution in molecular biology enabled the cloning— precise molecular characterization—of the genes that encode GPCRs and the proteins’ precise biochemical structures.
In the late 1990s, improved robotics and automation made it possible to screen enormous numbers of compounds against individual GPCRs, which accelerated drug discovery.
GPCRs are now considered leading targets for most major diseases, including neurological and psychiatric disorders, but targeting GPCRs in the brain is not easy. This entails designing a drug that can efficiently penetrate into the brain, then interact with only the targeted GPCR—and do so in a way to exert a beneficial effect while avoiding side effects mediated by actions in the brain as well as periphery. These are technical hurdles that have hindered brain-focused medications development. However, exciting new breakthroughs in understanding the structure of GPCRs promise to reinvigorate central nervous system (CNS)-focused drug discovery.
It is now possible to bombard GPCRs with X-rays and electrodes…
…to generate high-resolution pictures of these receptors in action.
A major barrier to developing new GPCR drugs is the remarkably small size of these proteins. To put their size into context, they are about 75 times smaller than a single wavelength of blue light (6 vs. 450 nm, respectively), making them difficult to visualize. Technological advances now make it possible to bombard GPCRs with X-rays and electrons to generate high-resolution pictures of these receptors in action.
Daniel Wacker, PhD, Assistant Professor of Pharmacological Sciences, and Neuroscience, and other FBI scientists can now capture exquisitely detailed 3D photographs of drugs bound to GPCRs. We currently have more than 400 such pictures that are yielding astonishing insights.
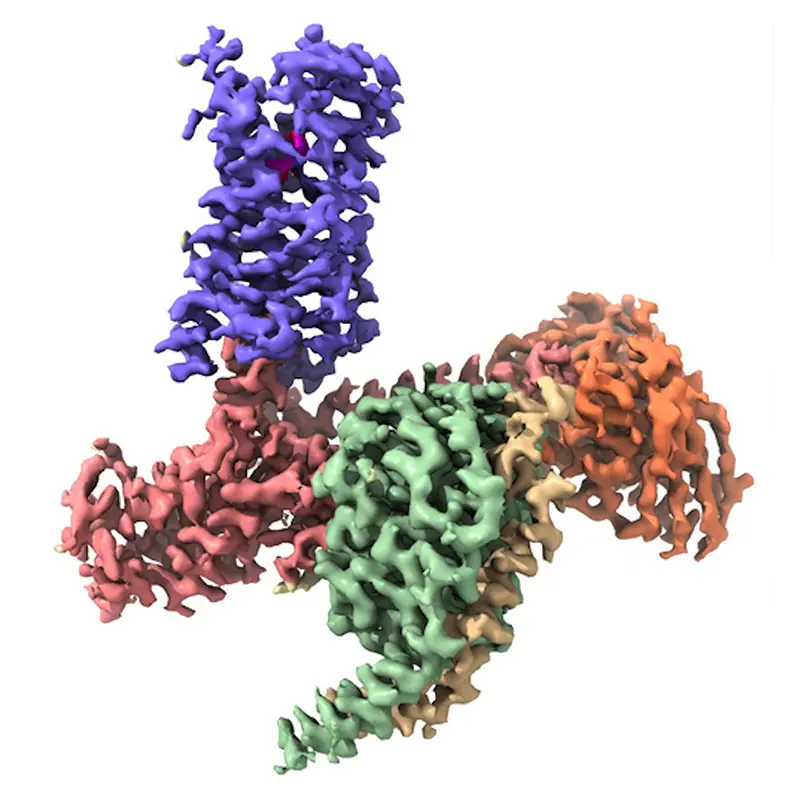
Figure 1. CryoEM Structure of the 5-HT2A Serotonin Receptor Signaling Complex Bound to the Psychedelic NBOH. Receptor, Gα, Gβ, Gγ, Fab, and NBOH are shown in purple, salmon, green, yellow, orange, and magenta, respectively. Credit: Daniel Wacker, PhD
In a flurry of seminal studies over the past decade, we have learned exactly how brain acting medications, such as opioid and antipsychotic drugs, bind to distinct, targeted GPCRs to cause their functional effects. We also better understand how illicit drugs such as psychedelics (Figure 1) act on GPCRs to alter brain physiology.
This is exciting because it could lead to entirely new classes of CNS medications derived from psychedelics but free from the mind-altering properties of these drugs. Having atomic scale blueprints of GPCRs is a windfall for medicinal chemists, who can now make precise alterations to compounds based on their new understanding of how such compounds physically interact with GPCRs. For instance, Jian Jin, PhD, and his colleagues at Mount Sinai (see related article here) have used structural insights into GPCRs to design new classes of compounds that may ultimately evolve into novel treatments for brain disorders.
Having atomic scale blueprints of GPCRs is a windfall…
…helping us make precise alterations to compounds...
Better snapshots of GPCRs may also help to reduce the negative side effects of existing medications. Often these side effects are caused by compounds interacting with GPCRs in an “unnatural” way that differs from how the receptor normally functions.
High-resolution 3D images of GPCRs trapped in conformations that are either beneficial or detrimental will help chemists to design new drugs with maximized therapeutic actions and minimized side effects. High-resolution images of GPCRs are also being used by computational neuroscientists to “virtually” discover new drugs. These pioneering scientists are applying machine learning and other artificial intelligence (AI) approaches to screen hundreds of millions of compounds, a scale impossible to achieve in the “real world,” to identify those most likely to physically interact with GPCRs.
The possibility of “next-generation” analgesics…
…with reduced addiction liability.
Mount Sinai scientists are playing leading roles in this revolutionary new way of designing drugs. For example, Marta Filizola, PhD, Dean of the Graduate School of Biomedical Sciences, has used innovative computational technologies to discover new classes of compounds that target opioid receptors, which may represent “next-generation” analgesics with reduced addiction liability (see related article here).
These thrilling developments at Mount Sinai highlight how structural studies have transformed GPCR drug discovery, and we are excited to apply these new methodologies and insights to deliver desperately needed new treatments for brain disorders that are both safe and effective.