The LBP study population is composed of individuals undergoing elective deep brain stimulation (DBS) surgery for neuropsychiatric conditions, including Parkinson’s disease, obsessive compulsive disorder, essential tremor, dystonia, Tourette syndrome, or treatment-resistant depression. An unprecedented quantity and diversity of data are collected from each LBP participant, which is used to characterize brain function from the level of single molecules in single cells all the way to determining the traits that make us human—thoughts, memories, emotions, and behaviors.
Learn more about these efforts in the following Q&A, where we highlight the work of Alexander G. Charney, MD, PhD, and Brian H. Kopell, MD, Co-Directors of the Living Brain Project.
Why is the LBP unique compared to other studies of the human brain?
Most of what is understood about the human brain comes from animal models, postmortem human brains, or limited studies on living human subjects in which the brain of each individual is characterized only using a small set of the tools in the clinical neuroscience toolkit—including clinical observation, neuropharmacology, neuroimaging, surface neurophysiology such as electroencephalography (EEG), and neuromodulation. The novelty of the LBP is that it utilizes the full clinical neuroscience toolkit, but adds to it the molecular and cellular characterization of living human brain tissue, which together provide a multifaceted view into the brain of each study participant.
The LBP has generated the largest known collection of brain specimens obtained from living individuals, along with paired blood and skin specimens. By creating a multidimensional dataset sourced from the same individual’s brain, the LBP is uniquely positioned to provide revelations that cannot be addressed using other study designs. For example, we can learn which gene and protein expression patterns in a person’s brain are active when the person experiences a particular emotion.
How is the DBS procedure different in the LBP?
Over the course of a routine DBS procedure, an area of the dorsolateral prefrontal cortex (DLPFC) is first cauterized to allow an electrode to be implanted deep in an individual’s brain, with a different site targeted for a given neuropsychiatric disorder. In the modified DBS procedure used for the LBP, the equivalent amount of living brain tissue around where the electrode enters the cortical surface is surgically removed, instead of being totally eliminated through cauterization. The total amount of tissue lost during the DBS procedure when a biopsy is performed is comparable to the amount of tissue lost during the DBS procedure with cauterization when a biopsy is not performed.
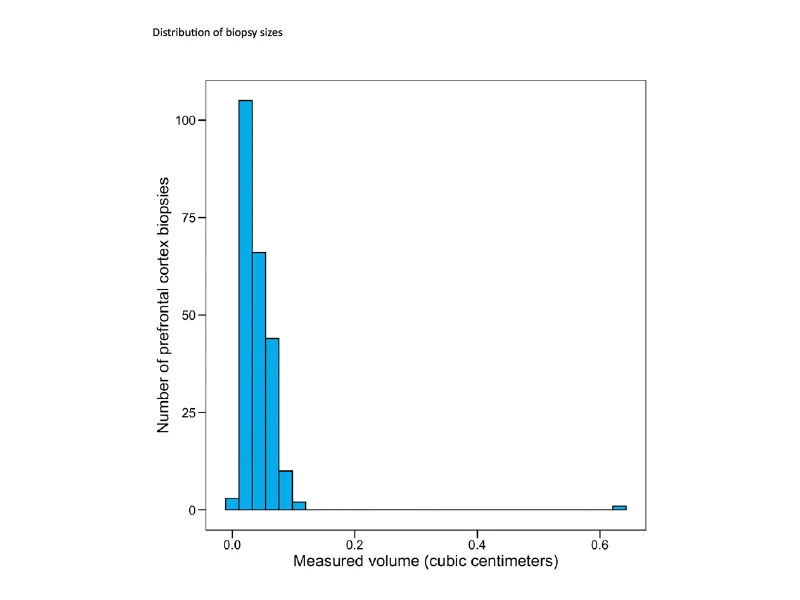
Figure 1. Histogram showing the distribution of the size of prefrontal cortex biopsies for the Living Brain Project. The mean volume of the biopsies is ~0.043 cm3. Figure source
What has been learned to date from the LBP?
The first 10 years of the LBP were focused on identifying molecular relationships between living and postmortem brain tissue, a critical investigation because the use of postmortem brain tissue is widespread in neuroscience even though it is unknown how living and postmortem brain tissue differ from one another.
Postmortem tissue is collected from individuals after death from disparate causes, with typically 6 to 18 hours passing between death and tissue collection. Not surprisingly, the LBP investigation identified widespread differences between living and postmortem tissue, painting a picture that suggests the molecular milieu of the brain is unsurprisingly dysregulated in the postmortem state (see research here). As just one example, a recent study demonstrated that RNA is extensively edited from adenosine to inosine in the postmortem brain, changes not seen in living brain tissue and likely caused by inflammatory- and hypoxia-related pathways that are activated after death.
The LBP has succeeded in linking molecular data, such as transcriptomic and proteomic analyses, from living brain samples to structural features obtained by MRI of the brains of the same individuals (see Figure 2). Parallel efforts are identifying sets of genes, whose expression levels correlate with neuronal firing in the brain while study participants played a simple computer game. Additionally, LBP studies have identified how dopamine and other neurotransmitter systems activate in our brains when we are making social decisions, and have contributed to the development of new technologies for studying how synapses in the brain work and how to deliver gene therapy to human brain cells.
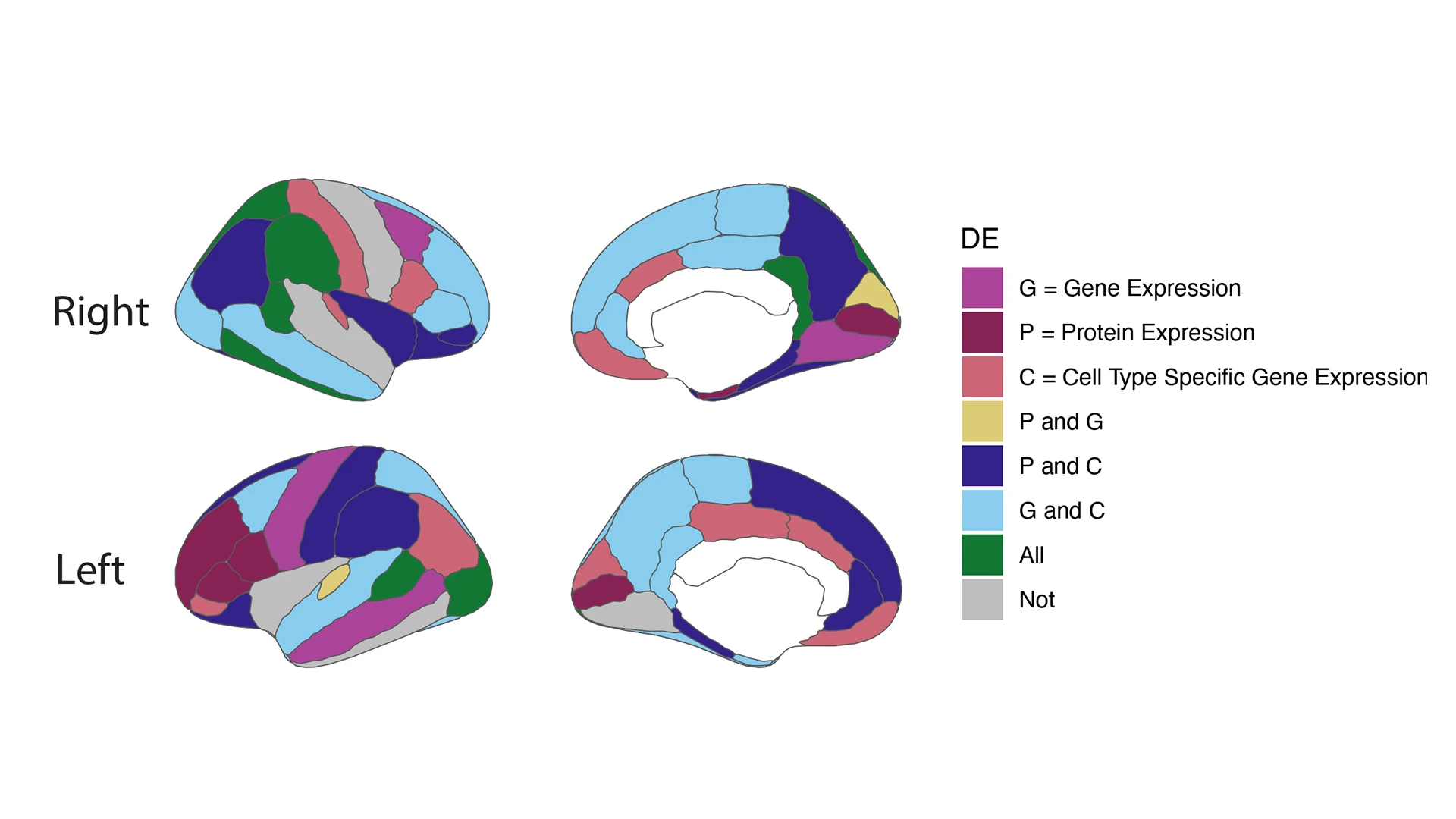
Figure 2. Gene and protein expression correlate with features of brain structure in the Living Brain Project.
RNA sequencing (RNAseq) and proteomic analyses were performed on living brain biopsies. Differential expression (DE) signatures between gene and protein expression in the prefrontal cortex (PFC) show association to different structural features taken from structural MRI (sMRI). Left panel shows right (top) and left (bottom) cortical hemispheres, illustrating several regions of interest (ROIs) in the DK (Desikan-Killiany) brain atlas. Right panel shows right (top) and left (bottom) subcortical hemispheres and several ROIs in the DK atlas. Features with significant DE genes (pi1 > 0.05) are colored and defined by the legend: G = gene expression (bulk RNAseq); P = protein expression (mass spectrometry); C = cell-type-specific gene expression (single nucleus RNAseq). White denotes subcortical features that are not included in the measurements. Credit: Anina Lund, Neuroscience PhD student
What Is the future of the LBP?
The LBP represents a pioneering new framework to study how our brains work. The vast amounts of data that are now being generated continuously by the LBP promise to allow researchers to develop novel diagnostic tests and more effective therapeutics that target specific genes in a precise manner to treat neurological and mental illnesses.
Featured
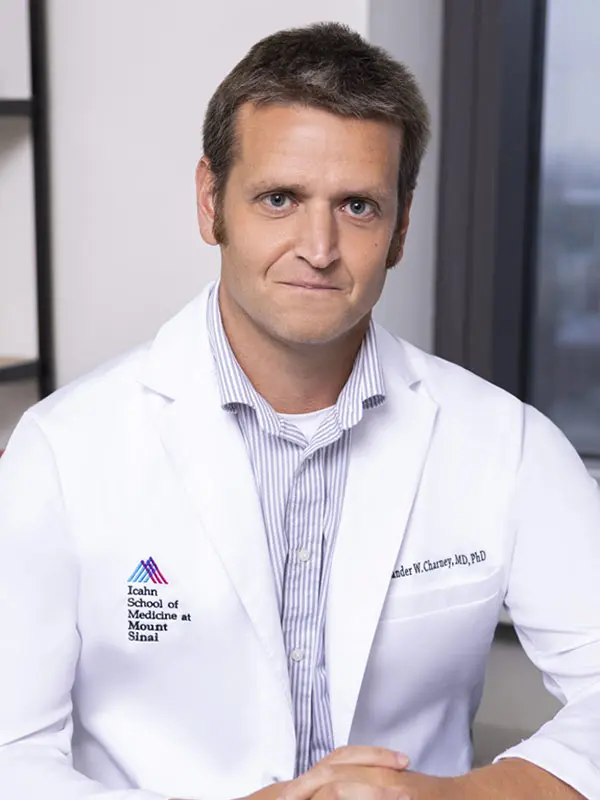
Alexander G. Charney, MD, PhD
Associate Professor of Psychiatry, Genetics and Genomic Sciences, Neuroscience, and Neurosurgery, and Director of the Charles Bronfman Institute for Personalized Medicine
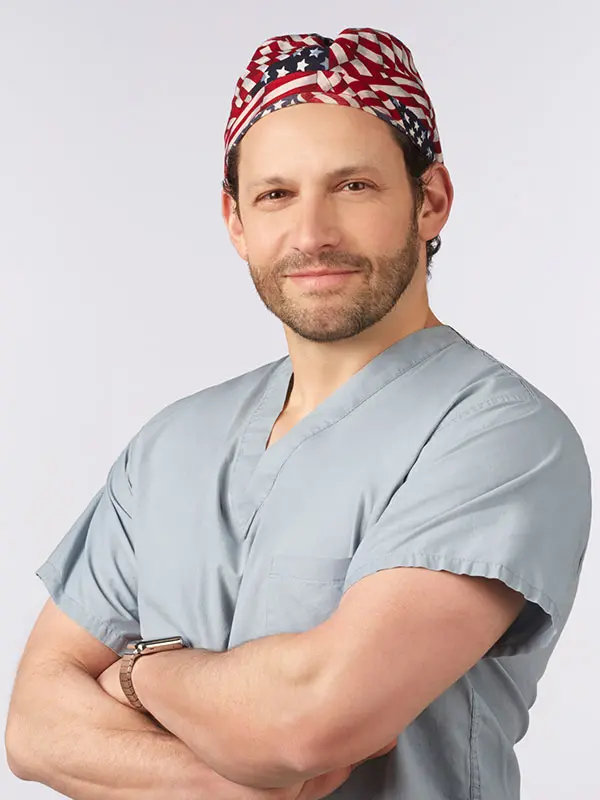
Brian H. Kopell, MD
Professor of Neurosurgery, Neurology, Psychiatry, and Neuroscience, and Director of the Center for Neuromodulation